Introduction:
In the last few decades, the field of computing has made monumental strides, with digital computers advancing from room-sized machines to the sleek laptops and smartphones we use today. However, as we approach the physical limits of classical computing, an exciting new frontier has emerged: quantum computing. While quantum computing is still in its early stages, its potential to revolutionize industries, particularly the tech industry, has generated significant excitement. But the burning question remains: Can quantum computing completely disrupt the tech industry in the coming years?
Quantum computing leverages the principles of quantum mechanics to process information in fundamentally different ways compared to classical computers. With the ability to perform calculations at exponentially faster speeds and solve problems deemed intractable by classical systems, quantum computers hold the promise of solving complex problems that could reshape entire sectors, including cybersecurity, artificial intelligence, medicine, and materials science.
This article will explore the current state of quantum computing, its potential to disrupt various areas of technology, the challenges it faces, and whether it can truly bring about a revolution in the tech industry within the next few years.
1. Understanding Quantum Computing: A Brief Overview
Quantum computing represents a drastic departure from classical computing. To understand its potential for disruption, it is crucial to grasp the basics of how quantum computers differ from traditional ones.
1.1. Classical vs. Quantum Computers
Classical computers process information in binary form—using bits, which are either 0 or 1. These bits are the smallest unit of information and form the foundation for all computations. When combined in large numbers, these bits perform a vast array of operations, but their capacity is ultimately limited by their binary nature and the physical limitations of hardware.
In contrast, quantum computers use qubits, which are quantum bits that can exist in multiple states simultaneously due to the principles of superposition and entanglement. A qubit can represent both 0 and 1 at the same time, which allows quantum computers to perform many calculations in parallel. Additionally, quantum entanglement allows qubits to be interconnected, meaning the state of one qubit can depend on the state of another, regardless of the distance between them.
This fundamentally different way of processing information means that quantum computers can solve certain problems much faster than classical computers, particularly those involving vast datasets and complex algorithms.
1.2. Quantum Mechanics in Action
Quantum computing relies on the principles of quantum mechanics, which govern the behavior of particles at the smallest scales. Some of these principles include:
- Superposition: Unlike classical bits, qubits can be in a state of 0, 1, or both at the same time. This enables quantum computers to explore multiple solutions simultaneously, drastically speeding up computation.
- Entanglement: Qubits that are entangled can influence each other, even across large distances. This property allows quantum computers to process information in ways that are impossible for classical computers.
- Quantum Interference: Quantum computers use interference to amplify the correct solutions to a problem and cancel out incorrect ones, further speeding up computations.
While these principles sound abstract, they form the backbone of quantum computing’s power and potential.
2. Quantum Computing’s Potential to Disrupt the Tech Industry
Quantum computing’s ability to solve problems exponentially faster than classical systems makes it a potentially transformative technology across various sectors of the tech industry. Let’s examine the areas where quantum computing could create the most disruption.
2.1. Cybersecurity and Cryptography
One of the most anticipated impacts of quantum computing is in the realm of cryptography, particularly the cryptographic protocols that protect modern communication. Many of the encryption algorithms we rely on today, such as RSA and ECC (Elliptic Curve Cryptography), are based on mathematical problems that are incredibly difficult to solve using classical computers. However, quantum computers could crack these encryption methods with ease using Shor’s algorithm, which allows for the factoring of large numbers in polynomial time.
This presents a potential existential threat to data privacy and security, as hackers could exploit quantum computers to break into encrypted systems. In response, there is a growing push toward post-quantum cryptography, which aims to develop encryption methods that are resistant to quantum attacks. Still, this area is in its early stages, and the actual deployment of quantum computers capable of breaking current cryptographic standards is likely still a few years away.
2.2. Artificial Intelligence and Machine Learning
Quantum computing has the potential to significantly enhance artificial intelligence (AI) and machine learning (ML) algorithms. Classical AI relies heavily on data processing and optimization, which can be time-consuming and computationally expensive. Quantum computers, with their ability to handle exponentially larger datasets and perform parallel computations, could significantly speed up the training of AI models, leading to faster and more accurate predictions.
For example, quantum computing could be applied to optimization problems, which are central to fields like logistics, finance, and drug discovery. Quantum algorithms could find optimal solutions to problems like supply chain management, portfolio optimization, and resource allocation much more efficiently than classical computers.
In machine learning, quantum computers could process and analyze large datasets in ways that would be infeasible for classical systems. This could lead to breakthroughs in areas like image recognition, natural language processing, and autonomous systems.
2.3. Materials Science and Drug Discovery
One of the most promising applications of quantum computing lies in materials science and pharmaceuticals. Many of the challenges in these fields involve understanding the interactions between atoms and molecules, which is an incredibly complex problem for classical computers. However, quantum computers can model the behavior of molecules and materials at the quantum level, enabling researchers to simulate chemical reactions and predict the properties of new materials with unprecedented accuracy.
For instance, quantum computers could be used to discover new materials for batteries that are more efficient or to develop new drugs by simulating the interactions between molecules. In drug discovery, quantum computing could revolutionize the development of personalized medicine by allowing researchers to simulate how different compounds interact with the human body on a molecular level, drastically speeding up the process.
2.4. Optimization and Logistics
Quantum computing could also bring about major improvements in optimization problems, which are present in numerous industries, including transportation, supply chain management, and manufacturing. Classical computers are often forced to use approximation methods to solve optimization problems, but quantum computers, with their parallel processing capabilities, can explore a vast number of possibilities simultaneously and find optimal solutions more efficiently.
This could result in breakthroughs in logistics, such as optimizing delivery routes for transportation companies, minimizing production costs for manufacturers, or improving traffic management in urban areas. With the ability to handle highly complex and dynamic systems, quantum computing could optimize entire supply chains and distribution networks in real time.
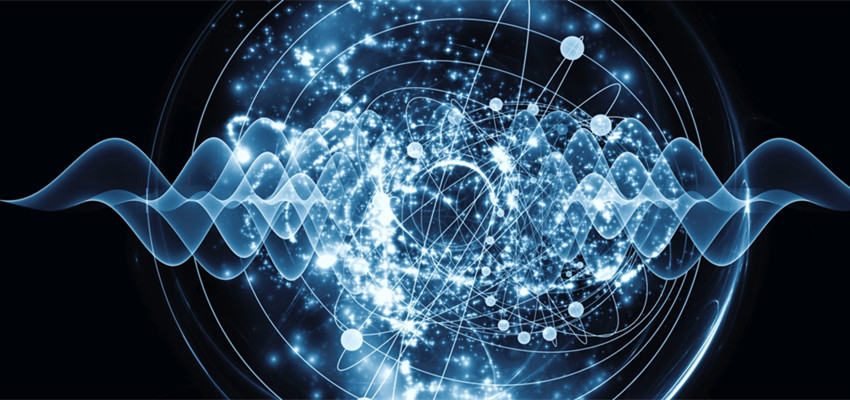
3. Challenges to the Widespread Adoption of Quantum Computing
While the potential for quantum computing to disrupt the tech industry is enormous, several challenges must be overcome before it can achieve widespread adoption.
3.1. Technological Hurdles
Quantum computers are extremely delicate. Qubits are highly sensitive to external factors like temperature, electromagnetic radiation, and even small vibrations. Maintaining a quantum state (known as coherence) long enough to perform meaningful calculations is a significant challenge. Current quantum computers require ultra-low temperatures to operate, and even small environmental changes can disrupt their calculations.
Despite advancements in quantum error correction and other techniques, quantum computers are still in the early stages of development. Most quantum computers today are still noisy and prone to errors, which limits their practical use for solving real-world problems.
3.2. Scalability
Quantum computers are still in their infancy, and the number of qubits that can be effectively utilized is relatively small. To truly unlock the power of quantum computing, scientists need to scale up quantum systems and improve the reliability of qubit interactions. Current quantum computers are far from being able to handle the scale of problems that could result in true disruption in industries like AI or cryptography.
Scalability remains one of the most significant obstacles to the widespread use of quantum computing, and researchers are working tirelessly to overcome it. However, it could take several more years or even decades before we have large-scale, fault-tolerant quantum computers that can solve complex, real-world problems.
3.3. Cost and Accessibility
Building and maintaining a quantum computer is incredibly expensive. The hardware requires advanced facilities, such as cryogenic systems to keep the quantum processors at extremely low temperatures. Additionally, the expertise required to develop and operate quantum computers is highly specialized.
For quantum computing to become widely accessible, the cost of developing and maintaining these machines must come down, and the software tools and infrastructure must become more user-friendly. Currently, only a few major tech companies, such as IBM, Google, and Rigetti, have the resources to invest in quantum computing research and development.
4. What Does the Future Hold for Quantum Computing?
Given the immense potential and challenges surrounding quantum computing, it’s difficult to predict exactly when it will disrupt the tech industry in a significant way. However, a few things are clear:
- Incremental Progress: While fully realized quantum computers are still some years away, we are likely to see incremental progress in quantum computing capabilities over the next few years. Hybrid systems that combine classical and quantum computing may provide useful solutions for certain problems in the interim.
- New Paradigms in Computing: As quantum computing matures, it could lead to the development of new computing paradigms and software tools designed to harness quantum capabilities. This could drive new industries and open up previously unimaginable opportunities.
- Collaboration and Innovation: Governments, tech companies, and academia are heavily investing in quantum computing research. The collaborative effort to address the challenges of quantum computing will likely accelerate progress and help shape the future of the technology.
Conclusion:
Quantum computing has the potential to disrupt the tech industry by solving problems that were previously deemed insurmountable for classical computers. Its applications in cybersecurity, AI, materials science, and optimization are poised to revolutionize multiple sectors. However, significant technological and scalability challenges must be addressed before quantum computing can fully realize its disruptive potential.
While we may not see quantum computing completely overhaul the tech industry in the next few years, incremental advancements will likely pave the way for more profound changes in the coming decades. The future of quantum computing is undoubtedly exciting, and its eventual impact will be felt across industries, reshaping the landscape of technology as we know it.